Oil well pipes are essential components in oil and gas exploration and development; however, corrosion remains a critical global issue. This study provides an overview of current research on oil well pipe corrosion in China, focusing on two key aspects: service environments and corrosion mechanisms. It reviews research on corrosion in oil fields across Northeast, North, and Northwest China, with particular attention to CO₂ corrosion, H₂S corrosion, and stress corrosion mechanisms. The study emphasizes the need for tailored corrosion protection measures based on the specific service environments of oil well pipes. Furthermore, it highlights the importance of further research into non-API pipes, bimetallic composite pipes, high-alloy pipes, and non-metallic pipes.
Fossil fuels, including coal, oil, and natural gas, continue to dominate global energy consumption. As demand rises, China has made becoming an energy powerhouse a priority, opening new opportunities for oil and gas exploration. With the expansion of oil and gas extraction, the corrosion of oil well pipes has become a significant challenge. Research indicates that approximately 20,000 oil well pipes in China’s Daqing, Changqing, and other oil fields corrode annually. The corrosion rate increases by 10% each year as service time extends, leading to considerable economic losses and posing safety risks in oil fields.
In China, the annual consumption of oil well pipes exceeds 2 million metric tons, with associated costs surpassing 200 billion RMB. As demand increases, oil and gas extraction is shifting toward high-pressure, high-temperature, and highly corrosive environments, often with complex geological conditions. In these challenging operations, corrosion resistance is critical for ensuring the durability and functionality of oil well pipes.
Yuze Li et al. investigated the corrosion fatigue behavior of 0.6Zr3Mo titanium alloy oil well pipes in hydrochloric acid gel at varying concentrations. Their study found that corrosion fatigue life decreased as acid concentration increased. Specifically, as the concentration of hydrochloric acid gel rose from 1% to 20%, the corrosion fatigue life declined in a linear manner. At low acid concentrations (1%), the fracture morphology of the titanium alloy resembled that observed in normal environments, with a single crack source and cleavage planes in the crack propagation zone. At higher concentrations (15%–20%), numerous pits appeared in the crack initiation zone, and cracks originated at multiple initiation sites. The crack propagation zone exhibited both primary and secondary cracks. The combined effects of cyclic loading and exposure to hydrochloric acid accelerated crack growth, leading to a significant reduction in the corrosion fatigue life of the titanium alloy. While research on oil well pipe corrosion is diverse, it remains fragmented. This study reviews the latest developments in corrosion research, focusing on two key aspects: service environments and corrosion mechanisms. The objective is to provide valuable insights to guide future research in this field.
China possesses rich oil reserves, primarily located in the Northeast, North China, and Northwest regions. The Tarim Oilfield, situated in the Taklimakan Desert of western Xinjiang, is characterized by a high concentration of CO₂, which increases the susceptibility of oil well pipes to corrosion.
Jiangbo Song et al. examined the corrosion resistance of two oil well pipe materials, L80-13Cr and N80Q (34Mn6 steel), in the Tarim Oilfield environment. Figure 1 presents the surface morphology of the samples after immersion corrosion. The results show that N80Q exhibits a higher corrosion rate in the liquid phase compared to the gas phase, while L80-13Cr maintains a low corrosion rate in both phases. As temperature increases to 100°C, both L80-13Cr and N80Q experience higher corrosion rates, with the rate increasing as the CO₂ partial pressure rises. These findings confirm that L80-13Cr oil well pipes are well-suited for the harsh conditions of the Tarim Oilfield, exhibiting superior corrosion resistance.
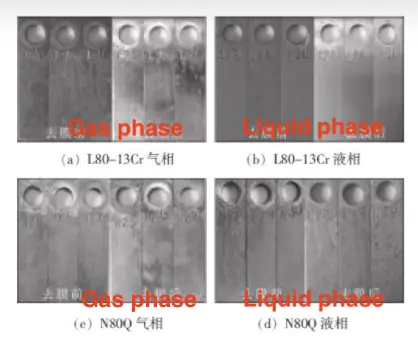
(a) L80-13Cr gas phase
(b) L80-13Cr liquid phase
(c) N80Q gas layer
(d) N80Q liquid phase
Figure 1: Surface morphology of sample after immersion corrosion
Haiyang Du et al. investigated the corrosion behavior of four types of casing materials (N80, 1Cr, 3Cr, and 13Cr) under the combined effects of CO₂ and H₂S in an oil field in the Bohai Sea. Their study found that carbon steel exhibited groove corrosion in a CO₂-only environment, minor pitting corrosion at low H₂S concentrations, and uniform corrosion under other conditions. Moreover, low concentrations of H₂S were found to mitigate CO₂-induced corrosion. Xiangzhi Jin et al. examined the corrosion behavior of N80 oil well pipe strings in dehydrated oil fields exposed to produced water. The study identified three types of corrosion: uniform corrosion, microbial corrosion induced by sulfate-reducing bacteria (SRB), and corrosion due to acidic gases such as CO₂ and H₂S. In certain areas, dense scaling formed, providing a protective effect against further corrosion. The study recommended the use of a 150 mg/L HSJ-02 corrosion inhibitor to mitigate pipe column corrosion. For oil wells experiencing severe corrosion, a combination of lined tubing, anti-corrosion rods, and double-valve anti-corrosion pumps should be implemented. Yi Yu et al. studied the corrosion behavior of TN110CR13M oil casing in an offshore oil and gas well under the following test conditions: a temperature of 129.5°C, CO₂ partial pressure of 2.75 MPa, and H₂S partial pressure of 0.26 kPa. The results demonstrated that the oil casing exhibited strong corrosion resistance, with an average corrosion rate of only 0.0188 mm per year. After 30 days of exposure, a Cr-containing corrosion product film formed on the surface, displaying a complete structure, high density, and enhanced corrosion resistance, offering further protection.
The deep layers of a gas field are characterized by high temperature, high pressure, low mineralization, and low H₂S content, resulting in a challenging environment with significant material loss due to corrosion but relatively low susceptibility to cracking. When selecting oil well pipes, it is essential to account for both corrosion-induced material loss from high CO₂ partial pressures and the potential for stress corrosion cracking from H₂S exposure. To address these concerns, Xuesong Xing et al. studied the corrosion behavior of Si3Cr, super duplex stainless steel 2507, and nickel-based alloy 2535 for oil casing under simulated field conditions. The results indicated that carbon steel did not experience stress corrosion cracking under these conditions but exhibited a high corrosion rate. Si3Cr showed no stress corrosion cracking, with corrosion rates of 0.0399 mm per year under middle-layer well conditions and 0.1633 mm per year under deep-layer well conditions. Both super duplex stainless steel 2507 and nickel-based alloy 2535 demonstrated low susceptibility to stress corrosion cracking, with corrosion rates of 0.0122 mm per year and 0.0083 mm per year, respectively.
China’s oil fields are widely distributed, with varying service environments. For instance, the Zhongyuan Oilfield has relatively high mineralization, ranging from 10×10⁴ to 16×10⁴ mg/L. The wastewater in this field contains high levels of divalent iron, as well as sulfate-reducing and saprophytic bacteria, with concentrations ranging from 10³ to 10⁴/mL. The pH is typically low, averaging around 6. Annually, over 60 oil wells are decommissioned due to casing corrosion. In the Tahe Oilfield, the primary cause of oil well pipe failure is excessive dissolved oxygen content. Given the diverse service environments and corrosion types in each oilfield, it is essential to conduct specific analyses and select targeted materials for oil well pipes to enhance their service life.
CO₂ corrosion refers to the corrosion caused by the presence of CO₂ and water in the pipe. Oil and gas wells often contain high concentrations of CO₂ during production. When CO₂ dissolves in produced or condensate water, it forms H₂CO₃ and CO₃²⁻, leading to electrochemical corrosion of steel. CO₂ corrosion is a form of dehydrogenation polarization corrosion and is often more severe than strong acid corrosion at the same pH level. In addition to being influenced by the depolarization reaction rate, its corrosion is also affected by the formation and stability of any protective film on the metal surface.
Yanming Liu studied the corrosion behavior of super 13Cr oil well pipes in a simulated CO₂ corrosion environment. The study found that as temperature and Cl⁻ concentration increased, the uniform corrosion rate of super 13Cr increased, peaking at 0.0164 mm per year at 150°C. However, the corrosion rate remained well below 0.1 mm per year. As temperature rose, partial corrosion of super 13Cr also increased, with a maximum partial corrosion rate of 1.1049 mm per year at 150°C. The uniform corrosion rate of super 13Cr first increased and then decreased with rising CO₂ partial pressure. The passivation film on the material’s surface primarily consists of amorphous Cr₂O₃. Hengmei Feng et al. studied the corrosion behavior of super 13Cr steel using high-temperature, high-pressure corrosion coupon tests and electrochemical tests at 141°C and a CO₂ partial pressure of 27.9 MPa. The study found that super 13Cr undergoes general corrosion, with a uniform corrosion rate of 3×10⁻³ mm per year. Electrochemical tests showed that the self-corrosion potential (-0.785V) and pitting potential (-0.301V) of 13Cr stainless steel were significantly more negative than those of super 13Cr stainless steel (-0.580V, -0.139V), with a higher self-corrosion current density and greater pure current density. The results indicate that under high CO₂ partial pressure, super 13Cr meets the requirements for gas field oil casings, offering superior corrosion resistance and lower corrosion sensitivity compared to 13Cr stainless steel.
In summary, CO₂ is an associated gas in oil and natural gas extraction, and it corrodes pipes when it comes into contact with water and other substances. CO₂ corrosion is particularly common in oil casings, as CO₂ is the primary gas in pipelines during gathering and transportation. Factors such as temperature, CO₂ partial pressure, water content, and pressure all influence the corrosion rate.
H₂S corrosion refers to the corrosion caused by the presence of H₂S and water in the pipe. There are two primary mechanisms: electrochemical corrosion and hydrogen-induced damage. When H₂S dissolves in water, it becomes acidic, leading to electrochemical corrosion of the pipe, which causes wall thinning or partial perforation. Hydrogen atoms generated during corrosion are absorbed by the steel and accumulate at alloy defects, potentially causing brittleness, crack initiation, and fracture. H₂S corrosion is a complex process, often influenced by the interaction of multiple factors. Studies indicate that the factors influencing H₂S corrosion include partial pressure of H₂S, Cl⁻ concentration, pH, temperature, and flow rate of the medium. Fengqin Bi et al. investigated the corrosion behavior of P110 steel in NaCl solutions with varying pH values through full immersion corrosion experiments and static electrochemical testing. They analyzed the corrosion rate, morphology, and mechanism of P110 steel by examining weight loss data, electrochemical polarization curves, and scanning electron microscopy images. The results show that pitting corrosion occurred on P110 steel in NaCl solutions with varying pH values. When the solution pH exceeds 3, the corrosion rate decreases, pitting corrosion on the sample surface decreases, and uniform corrosion increases. Conversely, when the pH is below 3, the corrosion rate decreases, but pitting density increases. Yanke Zhang’s research demonstrates that temperature significantly accelerates the corrosion behavior of P110 steel in H₂S solutions. An increase in temperature promotes the dissolution of the anode and accelerates the formation of FeSi-x corrosion products at the cathode. At 30°C, an increase in H₂S concentration from 0 to 28,900 mg/L significantly aggravated the corrosion of P110 steel and enhanced the cathodic hydrogen evolution reaction. During the H₂S corrosion process, exfoliation of Fe/C caused varying degrees of pitting on P110 steel.
Stress corrosion cracking (SCC) includes a specific type known as sulfide stress corrosion cracking (SSCC), which refers to brittle fracture caused by the interaction between a corrosive medium and applied stress when metal materials are exposed to a sulfide environment under tensile stress. This damage results from the combined effects of applied stress and a corrosive environment, requiring three essential conditions: susceptible materials, an acidic environment, and tensile stress. Unlike conventional stress corrosion, which primarily relies on anodic dissolution, sulfide stress corrosion cracking mainly involves cathodic hydrogen charging. While the exact mechanism is not fully understood, hydrogen embrittlement is often cited as the cause of sulfide stress corrosion cracking.
Wanli Gao and colleagues studied the corrosion behavior of oil well pipes in the Lijiawan area of the Shuanghe Oilfield. They found that the chloride concentration in the oil field reached 14,189.30 mg/L, with corrosion in the area primarily caused by chloride stress corrosion and concentration corrosion due to high mineralization. Additionally, sulfur and its compounds can directly or indirectly cause varying degrees of corrosion to sewage treatment and reinjection equipment, while sulfate-reducing bacteria (SRB) may induce microbial corrosion.
Yameng Qi and colleagues investigated the resistance to sulfide stress corrosion cracking of 80SS, 90SS, and 110SS sulfur-resistant oil casings under CO₂ and H₂S composite conditions using constant load tests. The study found that under CO₂ and H₂S conditions, all constant load specimens of 80SS, 90SS, and 110SS sulfur-resistant oil casings fractured, with fracture times ranging from 202 to 800 hours. The fracture time decreased significantly with higher steel grades. At the same steel grade, an increase in CO₂ and H₂S gas content had minimal effect on the fracture time of 120SS. Under these conditions, all three grades of sulfur-resistant oil casings exhibited significant corrosion thinning. As the steel grade decreased, the average diameter thinning increased from 10% to 33%. The presence of CO₂ caused the pH of the solution to continuously decrease, eventually dropping below 2.85, as shown in Figure 2.
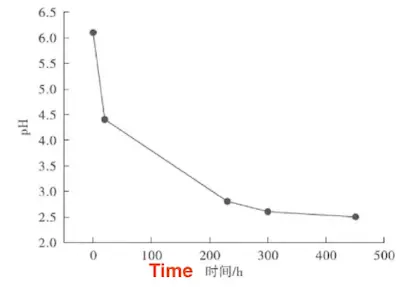
Figure 2: pH Value of O₂/H₂S = 1 Solution for 110SS at 66% SMYS vs. Corrosion Time
Oil exploration and development in China have been ongoing for many years, with varying degrees of corrosion affecting oil well pipes. These corrosion issues include CO₂ corrosion, H₂S corrosion, and stress corrosion, with cases where two or more types coexist. The selection of the most economical and environmentally friendly corrosion protection technology for oil and gas well pipes should be based on specific environmental and operational conditions, including pressure, temperature, and the presence of corrosive substances. As the challenges of oil and gas exploration evolve, there has been an increasing focus on the exploration and development of offshore oil and gas resources. Therefore, the corrosion and protection of offshore oil well pipes will be critical areas for future research. Additionally, further studies are needed on non-API pipes, bimetallic composite pipes, high-alloy pipes, and non-metallic pipes to improve the performance and durability of oil well casings under challenging conditions.